Control of structure, strain and chemistry: a route to designer fuel cell interfaces

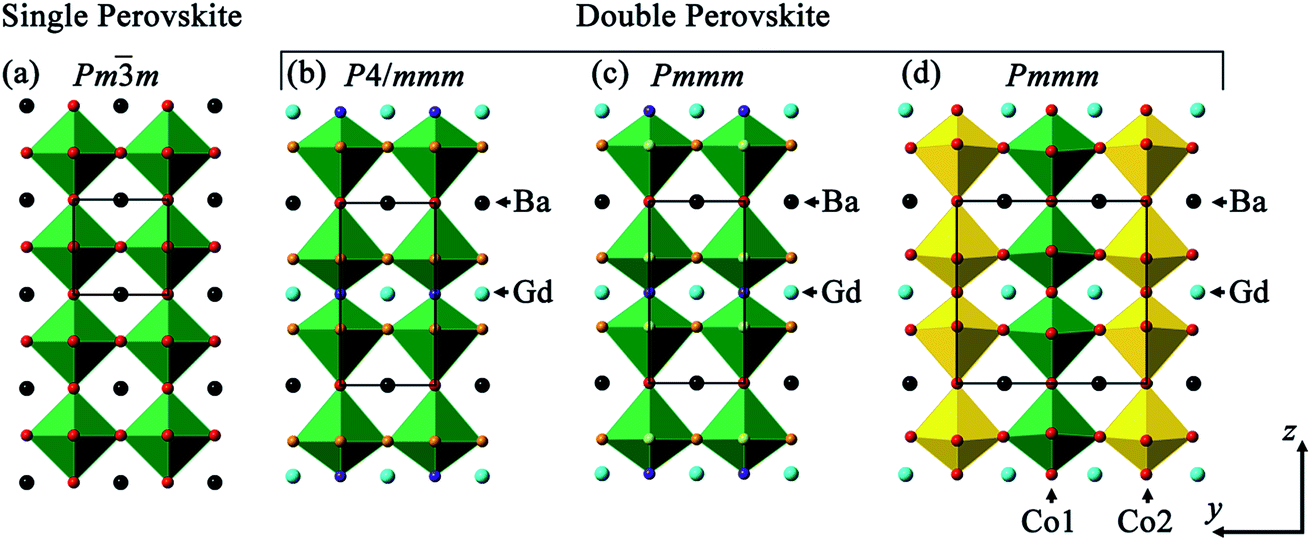
A fuel cell consists of three primary components: the air electrode, fuel electrode and ion transport electrolyte. The function of these components is primarily to carry current, reduce oxygen and oxidise a fuel. As these devices are typically constructed using traditional manufacturing techniques there is little control of the atomic scale processes that occur at the interfaces between each of these components. As the electrochemistry that controls the fuel cell operation is correlated with the structure and strain at the interfaces between the components and with the electrode/environment interfaces, a clear understadning of these processes at the atomic scale is essential if optimised, high performacne, low cost fuel cells are to be produced. In this work we use a complementary suite of advanced techniques, including X-ray photoelectron spectroscopy, Low energy ion scattering and crystal truncation rods to probe the structure of the interfaces, including buried interfaces, and link this with surface chemistry and fuel cell performance.
Project Funding: EPSRC
Selected Publications: Li et al. Chem Mater. (2019), Cavallaro et al. Sustain. Energy & Fuels (2018), Pramana et al. J. Mater. Chem. A (2018) , Harrington et al. PCCP (2017), Pramana et al. J. Am. Chem. Soc. (2016)
In situ probing of transition metal-oxide heterointerfaces for high-performance solid-state energy devices
The discovery of complex transition metal oxide (TMOs) heterostructures as multifunctional materials of striking flexibility had been a significant advancement in the solid-state ionics field. TMOs have distinctively different properties arising from the heterointerfaces, such as fast ion exchange/diffusion kinetics, catalysis, superconductivity, ferroelectricity and resistive switching. These phenomena emerge from the complex interactions between the lattice, charge, spin and orbital degrees of freedom. They are highly sensitive to external stimuli such as strain, chemical doping, and electric and magnetic fields. Notably, engineering the strain field across the heterointerfaces offers a new route to design high-performance solid-state energy devices including solid oxide cells and solid-state batteries.
Manipulating these heterointerfaces through design has become an effective tool to study such functionalities. Particularly, a lateral multilayer heterostructure design has been widely explored, highlighting superior electronic and ionic transport properties. For example, compared with either single-phase, cobaltite heterointerfaces, (LaSr)CoO3/(LaSr)2CoO4, exhibit 3 orders of magnitude faster oxygen surface exchange kinetics [1]. However, as the origin of these enhancements remains unclear, the optimisation of the performance and the incorporation of these systems in commercial devices remain limited. Two main reasons are hindering their research and development so far:
-
Limited instrumental resolutions compared to the nanometre length scale of the heterointerfaces,
-
Difficulty in investigating the chemical structure and reaction kinetics at the buried heterointerfaces in multilayer design.
​
In order to solve these problems, EU-funded INSPIRE project investigates two main objectives:
-
Use of self-assembled VAN electrode architectures (Figure 1a) as a simple, synthetic route to control the properties of heterointerfaces.
-
Quantitative analysis of the chemical, structural and microstructural features governing the ionic/electronic transport and degradation processes in heterointerfaces by using electrochemical analysis and a unique set-up of surface analysis techniques.
[1] Sase, M. et al. Solid State Ionics. 178, 1843-1852 (2008).

Project Funding: Marie Skłodowska-Curie Actions Individual Fellowship
Selected Publications: Celikbilek et al. ACS Appl Mater Inter (2020)